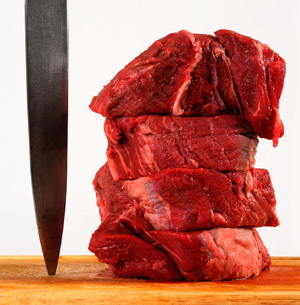
Introduction
In a recent article published last week in the prestigious Nature Medicine journal [1], researchers presented novel data on the pathogenesis of cardiovascular disease (CVD), arguing that it’s not the saturated fat (SFA) or cholesterol in your red meat that’s causing your arteries to clog; rather, it’s the carnitine. What’s more is that the media just can’t get enough of it. Indeed, both the New York Times and the Wall Street Journal (just to name a few) jumped on this story and put out articles with quotations from experts warning people against red meat consumption. While this type of alarmism is nothing new, especially with regards to red meat (remember last summer when red meat would just flat out kill you?), this new article is extremely interesting and does offer up a novel explanation for (part of) why red meat is constantly being associated with cardiovascular disease (CVD).
Nevertheless, in spite of the originality of this new paper and its potential implications for future health policy, we should remain critical of the paper’s content and not take giant leaps of faith in accepting new research findings as dogmatic fact (I’m looking at you vegans and vegetarians). Therefore, today’s goal is show you why you should not fear red meat, because it can, like all others foods, fit easily into anyone’s diet and impart beneficial effects on health: even heart-health!
Prevailing paradigm
It’s no news that the prevailing notion about red meat has been that its accompanying SFA and cholesterol are the primary driving forces behind the food’s association with CVD [2-5]. However, a recent meta-analysis refutes this assumption after showing no association between SFA and CVD [6]. While investigation into several other potential disease-causing mediators that commonly accompany red meat, such as salt [7] and heterocyclic compounds (you know, the char on your meat that gives it that “grill taste” you enjoy so much) [8] are proving to be less fruitful in the red meat-CVD debate – albeit salt is generating some interesting data with regards to autoimmune disease progression [9, 10] – today’s article looked at something no one had ever really considered before: carnitine.
Primer on carnitine
In order to save time, I have copied and pasted the following paragraph on carnitine from a previous article wherein I evaluated carnitine’s (null) effects on fat loss and performance. For those interested, you can see the full article here:
“Carnitine – named after the Latin word carnis, meaning ‘flesh’ – is a vitamin-like, water-soluble amine that can be obtained through dietary intake (for example: meat and milk) or by endogenous synthesis via S-adenosyl-methionine (SAM) and lysine in both the liver and kidneys. Almost all (~95-98%) of the bodily stores of carnitine are present in skeletal muscle and in the heart (with the remaining 2-5% in the liver, kidneys and plasma). Carnitine plays a pivotal role in both fat metabolism as well as carbohydrate metabolism […] Throughout most of the day (assuming you don’t sprint everywhere) the human body runs on a mixture of glucose, amino acids, and free fatty acids, with the majority of ATP coming from FFAs. However, in order to oxidize these FFAs (and yield energy in the form of ATP) each fatty acid must undergo a process called beta-oxidation. Beta-oxidation takes place within the matrix of the mitochondria of the cell. In order for the fatty acid to even make it into the matrix, it must rely on the help of carnitine to facilitate its transport in. Once inside, the fatty acids can undergo beta-oxidation and proceed to the Tricarboxylic acid (TCA) cycle and produce ATP.”
Furthermore, carnitine and its derivatives have shown huge promise in various pathological conditions, some of which happen to be to cardiovascular and heart disease [11-14]. While this may seem counterintuitive – even contradictory – to the published Nature Medicine article which argues that carnitine causes CVD, one has to remember that the physiology/biochemistry during a pathological condition is very different from that of a relatively healthy person. Indeed, the benefits of carnitine supplementation for certain cardiovascular and heart pathologies are due to the reduction in heart carnitine levels caused by the condition itself (usually the lack of oxygen to the heart, called ischemia) [13]. Therefore, it would not be wise to say that carnitine is contraindicated for those with heart disease because, in fact, supplementation with carnitine actually improves health under certain cardiovascular conditions. Like all things, nothing is ever black and white. So before I start getting ahead of myself, let’s actually examine the Nature article and see why carnitine might lead, or even (dare I say it) cause, cardiovascular and heart disease.
Biological rationale for carnitine’s role in CVD pathogenesis
The authors, in an attempt to unveil some earth-shattering mechanism for why red meat might be causing CVD, suggest that there could possibly be some type of microbe-dependent, diet-host mechanism that had previously been unexplored. In other words, perhaps the bacteria in our intestines are somehow contributing to CVD. Recent evidence in both human and animal models suggests a role for such a mechanism in a whole host of diseases, such as obesity [15-17] and type II diabetes [18]. Therefore, the idea for a similar rationale in CVD is not so farfetched.
In preliminary research, the authors identified a group of metabolites that were associated with CVD risk [19], wherein, upon further analysis, they found that the metabolite known as trimethylamine N-oxide (TMAO for short) was the primary candidate. Furthermore, the researchers also discovered that this metabolite happens to be a product of gut-dependent-choline metabolism, providing further evidence for a microbial role in CVD progression.
So how does carnitine fit into the picture? Well, carnitine is similar in structure to choline (they’re both trimethylamines) and therefore undergoes the same metabolism as choline (see figure below).
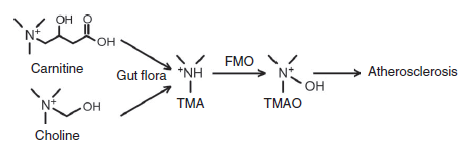
This in turn produces TMAO and increases plasma levels which could lead to CVD. While carnitine is present in other foods such as fish (a food commonly associated with improving CVD risk [20, 21]), the levels of carnitine are about 10-12 times higher in red meat [22]; potentially letting fish off the proverbial hook, so to speak. However, as it turns out, some types of fish are actually fairly high in TMAO [23]; lending less credence to the notion that only red meat (and not other forms of meat like fish) possibly leads to CVD.
Article findings
To keep things simple (because this article was a beast and I don’t feel like getting into every little methodological detail), I’m going to bullet point the major results. If you REALLY want to read the study, e-mail me and I’ll send it your way. The findings were as follows:
- Carnitine was associated with CVD
- Human gut microbes are required to form TMAO from carnitine
- Vegans and vegetarians produce much less TMAO than non-vegans, and this is primarily due to differences in gut bacteria composition
- A dose-dependent relationship between plasma levels of carnitine and CVD exists; however, TMAO was shown to be the driving force behind this association. Therefore, it is not the carnitine, per se, that potentially causes CVD. Rather, it’s TMAO.
- ApoE -/- (knockout) mice were shown to have a doubling of CVD progression when supplemented with carnitine or choline.
- TMAO inhibits cholesterol removal from peripheral tissues back to the liver in ApoE -/- mice. This, effectively, shifts the net balance towards cholesterol deposition and accumulation, which can progress to atherosclerosis and CVD/heart disease.
So, to briefly summarize, an association (not causation) between carnitine and CVD risk was established in humans, with subsequent rodent data to help provide the mechanistic justification as to why. However, as with all rodent data, there needs to be some reservation when trying to apply the findings to humans (Again, I’m looking at you vegans/vegetarians). ApoE -/- knockout mice have a genetic deletion that specifically causes them to develop dyslipidemia (high blood lipids) and atherosclerosis [24]. Indeed, even in the face of a regular chow, low cholesterol diet, these mice have blood cholesterol levels upwards of 400mg/dL (normal levels ~80mg/dL). This was more than sufficient to induce CVD, showing that plasma lipids do contribute to the pathogenesis of the disease. Therefore, it was shown that, in mice that already had CVD and severe dyslipidemia, a diet with supplemental carnitine exacerbated the disease; it did not cause it. Moreover, the levels of carnitine used to exacerbate the disease were beyond supraphysiological. The mice would have had to consume over 6lbs of red meat per day in order to reach the levels of carnitine they were supplemented with (~2g carnitine/day; the average cut of red meat contains ~70mg/100g [22]). This intake would be even greater for humans. Not realistic by any stretch of the imagination.
In the end, until subsequent research comes out involving more human-derived data, we just can’t translate preliminary and completely unrealistic rodent data into health policy-altering fact. Furthermore, there is substantial data in humans involving realistic intakes red meat that at least makes us think twice about red meat’s role in CVD. Indeed, this research shows actual improvements in traditional risk factors (like someone’s cholesterol levels (LDL and HDL) that do promote CVD) when beef is consumed alongside a reasonable diet (i.e. one with fruits and vegetables, etc.). While these studies do nothing to refute carnitine’s potential role in CVD progression, they do lend credence to the fact that red meat can be incorporated into a traditional heart-healthy diet which actually improves health. Whether or not carnitine/TMAO can exert their potential CVD-causing effects independent of an improved lipid profile and a realistic intake of red meat is far from established, and hard to believe. Obviously future research will need to address this concern.
Evidence to the contrary
Despite the craze after last week’s Nature Medicine article, the fact of the matter is that red meat can be incorporated into a diet that actually lowers one’s risk for CVD and heart disease, based on traditional risk factors, like total, LDL and HDL cholesterol levels. A non-exhaustive list of the research follows.
In 1994, Scott et al. [25] saw that, in free living subjects, a 5-week diet containing red meat had similar effects on lowering plasma total and LDL cholesterol when compared to a similar diet containing chicken. Five years later in 1999, Davidson and co. [26] also showed that, in free-living subjects consuming lean red meat, subjects lowered total and LDL cholesterol, as well as, raised HDL cholesterol to the same degree as those on a white meat diet. Fast forward to 2003, to quote the concluding remarks of Beauchesne-Rondeau et al. who examined the effects of beef, lean fish and poultry on lipoprotein profiles in hypercholesterolemic men (important line bolded) [27]:
“[W]ith respect to [heart disease] risk, an AHA diet with a high [polyunsaturated to saturated fat ratio] and high fiber content, regardless of the protein source, induced numerous favorable changes such as reductions in plasma total and LDL cholesterol and apo B, total and VLDL triacyl-glycerols, and total:HDL cholesterol in hypercholesterolemic men, and it overlapped the effects of protein sources on LDL apo B pre-viously observed in normocholesterolemic subjects.”
Finally, in a well-controlled study conducted last year, Roussell et al. [28] showed that, when incorporated into a well-balanced diet (again, plenty of fruits and vegetables) red meat can actually improve one’s heart health. This was evidenced by improvements in total and LDL cholesterol, as well as, plasma triglycerides. To quote the authors directly;
“[These] results […] provide convincing evidence that lean beef can be included in a heart-healthy diet that meets current dietary recommendations and reduces CVD risk.”
Concluding remarks
So, even with the incorporation of red meat into someone’s diet, one can definitely improve their lipid profile and reduce their risk for CVD and heart disease, as long as that diet is sensible and contains reasonable amounts of fruits and vegetables, etc. The big thing to keep in mind is that, while new research like this may seem compelling and interesting, it’s often wrought with the media’s biased, sensationalist coverage. It’s important to keep in mind the overall context of one’s diet when evaluating the individual food constituents and their effects on someone’s health. Red meat should not be feared or vilified. Rather, it should be eaten and enjoyed, in moderation, like all other foods.
There is still a lot to be learned about carnitine and its role in CVD. As we have seen, under certain pathological conditions supplemental carnitine and its derivatives can be beneficial to patients with previous heart complications. Furthermore, it has yet to be shown why foods like fish, which have been shown to have the detrimental metabolite TMAO, are not usually associated with CVD. Lastly, I want to also point out that physical activity and life style modifications have yet to be mentioned in this discussion. Given my fitness-oriented readership, I feel the need to at least briefly mention that weight-loss and physical activity both affect the composition of one’s gut microbiota. Indeed, a couple of the strains of bacteria that were shown to be associated with carnitine metabolism in the Nature article were actually shown to be reduced in another study which observed overweight individuals who lost weight and increased their physical activity [29].
So, while the masses may buy into the media’s frenzy about red meat being evil, I’ll be firing up the grill this weekend and enjoying a nice steak.
References
1. Koeth RA, Wang Z, Levison BS, Buffa JA, Org E, Sheehy BT, Britt EB, Fu X, Wu Y, Li L, et al: Intestinal microbiota metabolism of l-carnitine, a nutrient in red meat, promotes atherosclerosis. Nat Med 2013.
2. Wilson PW, Kannel WB, Silbershatz H, D’Agostino RB: Clustering of metabolic factors and coronary heart disease. Arch Intern Med 1999, 159:1104-1109.
3. Keys A: Coronary heart disease, serum cholesterol, and the diet. Acta Med Scand 1980, 207:153-160.
4. Micha R, Wallace SK, Mozaffarian D: Red and processed meat consumption and risk of incident coronary heart disease, stroke, and diabetes mellitus: a systematic review and meta-analysis. Circulation 2010, 121:2271-2283.
5. Bernstein AM, Sun Q, Hu FB, Stampfer MJ, Manson JE, Willett WC: Major dietary protein sources and risk of coronary heart disease in women. Circulation 2010, 122:876-883.
6. Siri-Tarino PW, Sun Q, Hu FB, Krauss RM: Meta-analysis of prospective cohort studies evaluating the association of saturated fat with cardiovascular disease. Am J Clin Nutr 2010, 91:535-546.
7. Bibbins-Domingo K, Chertow GM, Coxson PG, Moran A, Lightwood JM, Pletcher MJ, Goldman L: Projected effect of dietary salt reductions on future cardiovascular disease. N Engl J Med 2010, 362:590-599.
8. Hansen ES: International Commission for Protection Against Environmental Mutagens and Carcinogens. ICPEMC Working Paper 7/1/2. Shared risk factors for cancer and atherosclerosis–a review of the epidemiological evidence. Mutat Res 1990, 239:163-179.
9. Wu C, Yosef N, Thalhamer T, Zhu C, Xiao S, Kishi Y, Regev A, Kuchroo VK: Induction of pathogenic T17 cells by inducible salt-sensing kinase SGK1. Nature 2013.
10. Kleinewietfeld M, Manzel A, Titze J, Kvakan H, Yosef N, Linker RA, Muller DN, Hafler DA: Sodium chloride drives autoimmune disease by the induction of pathogenic T17 cells. Nature 2013.
11. Malaguarnera M: Carnitine derivatives: clinical usefulness. Curr Opin Gastroenterol 2012, 28:166-176.
12. Pauly DF, Pepine CJ: The role of carnitine in myocardial dysfunction. Am J Kidney Dis 2003, 41:S35-43.
13. Mingorance C, Rodriguez-Rodriguez R, Justo ML, Alvarez de Sotomayor M, Herrera MD: Critical update for the clinical use of L-carnitine analogs in cardiometabolic disorders. Vasc Health Risk Manag 2011, 7:169-176.
14. Serati AR, Motamedi MR, Emami S, Varedi P, Movahed MR: L-carnitine treatment in patients with mild diastolic heart failure is associated with improvement in diastolic function and symptoms. Cardiology 2010, 116:178-182.
15. Backhed F, Manchester JK, Semenkovich CF, Gordon JI: Mechanisms underlying the resistance to diet-induced obesity in germ-free mice. Proc Natl Acad Sci U S A 2007, 104:979-984.
16. Turnbaugh PJ, Gordon JI: The core gut microbiome, energy balance and obesity. J Physiol 2009, 587:4153-4158.
17. Turnbaugh PJ, Hamady M, Yatsunenko T, Cantarel BL, Duncan A, Ley RE, Sogin ML, Jones WJ, Roe BA, Affourtit JP, et al: A core gut microbiome in obese and lean twins. Nature 2009, 457:480-484.
18. Greiner T, Backhed F: Effects of the gut microbiota on obesity and glucose homeostasis. Trends Endocrinol Metab 2011, 22:117-123.
19. Wang Z, Klipfell E, Bennett BJ, Koeth R, Levison BS, Dugar B, Feldstein AE, Britt EB, Fu X, Chung YM, et al: Gut flora metabolism of phosphatidylcholine promotes cardiovascular disease. Nature 2011, 472:57-63.
20. He K, Song Y, Daviglus ML, Liu K, Van Horn L, Dyer AR, Greenland P: Accumulated evidence on fish consumption and coronary heart disease mortality: a meta-analysis of cohort studies. Circulation 2004, 109:2705-2711.
21. Whelton SP, He J, Whelton PK, Muntner P: Meta-analysis of observational studies on fish intake and coronary heart disease. Am J Cardiol 2004, 93:1119-1123.
22. Rigault C, Mazue F, Bernard A, Demarquoy J, Le Borgne F: Changes in l-carnitine content of fish and meat during domestic cooking. Meat Sci 2008, 78:331-335.
23. Yancey PH: Organic osmolytes as compatible, metabolic and counteracting cytoprotectants in high osmolarity and other stresses. J Exp Biol 2005, 208:2819-2830.
24. Pendse AA, Arbones-Mainar JM, Johnson LA, Altenburg MK, Maeda N: Apolipoprotein E knock-out and knock-in mice: atherosclerosis, metabolic syndrome, and beyond. J Lipid Res 2009, 50 Suppl:S178-182.
25. Scott LW, Dunn JK, Pownall HJ, Brauchi DJ, McMann MC, Herd JA, Harris KB, Savell JW, Cross HR, Gotto AM, Jr.: Effects of beef and chicken consumption on plasma lipid levels in hypercholesterolemic men. Arch Intern Med 1994, 154:1261-1267.
26. Davidson MH, Hunninghake D, Maki KC, Kwiterovich PO, Jr., Kafonek S: Comparison of the effects of lean red meat vs lean white meat on serum lipid levels among free-living persons with hypercholesterolemia: a long-term, randomized clinical trial. Arch Intern Med 1999, 159:1331-1338.
27. Beauchesne-Rondeau E, Gascon A, Bergeron J, Jacques H: Plasma lipids and lipoproteins in hypercholesterolemic men fed a lipid-lowering diet containing lean beef, lean fish, or poultry. Am J Clin Nutr 2003, 77:587-593.
28. Roussell MA, Hill AM, Gaugler TL, West SG, Heuvel JP, Alaupovic P, Gillies PJ, Kris-Etherton PM: Beef in an Optimal Lean Diet study: effects on lipids, lipoproteins, and apolipoproteins. Am J Clin Nutr 2012, 95:9-16.
29. Santacruz A, Marcos A, Warnberg J, Marti A, Martin-Matillas M, Campoy C, Moreno LA, Veiga O, Redondo-Figuero C, Garagorri JM, et al: Interplay between weight loss and gut microbiota composition in overweight adolescents. Obesity (Silver Spring) 2009, 17:1906-1915.